Clinical Impact of Genomic and Pathway Alterations in Stage I EGFR-Mutant Lung Adenocarcinoma
Article information
Abstract
Purpose
We investigated the clinical impact of genomic and pathway alterations in stage I epidermal growth factor receptor (EGFR)–mutant lung adenocarcinomas, which have a high recurrence rate despite complete surgical resection.
Materials and Methods
Out of the initial cohort of 257 patients with completely resected stage I EGFR-mutant lung adenocarcinoma, tumor samples from 105 patients were subjected to analysis using large-panel next-generation sequencing. We analyzed 11 canonical oncogenic pathways and determined the number of pathway alterations (NPA). Survival analyses were performed based on co-occurring alterations and NPA in three patient groups: all patients, patients with International Association for the Study of Lung Cancer (IASLC) pathology grade 2, and patients with recurrent tumors treated with EGFR–tyrosine kinase inhibitor (TKI).
Results
In the univariate analysis, pathological stage, IASLC grade, TP53 mutation, NPA, phosphoinositide 3-kinase pathway, p53 pathway, and cell cycle pathway exhibited significant associations with worse recurrence-free survival (RFS). Moreover, RPS6KB1 or EGFR amplifications were linked to a poorer RFS. Multivariate analysis revealed that pathologic stage, IASLC grade, and cell cycle pathway alteration were independent poor prognostic factors for RFS (p=0.002, p < 0.001, and p=0.006, respectively). In the grade 2 subgroup, higher NPA was independently associated with worse RFS (p=0.003). Additionally, in patients with recurrence treated with EGFR-TKIs, co-occurring TP53 mutations were linked to shorter progression-free survival (p=0.025).
Conclusion
Genomic and pathway alterations, particularly cell cycle alterations, high NPA, and TP53 mutations, were associated with worse clinical outcomes in stage I EGFR-mutant lung adenocarcinoma. These findings may have implications for risk stratification and the development of new therapeutic strategies in early-stage EGFR-mutant lung cancer patients.
Introduction
Complete surgical resection with mediastinal lymph node dissection remains the gold standard treatment for patients with early-stage non–small cell lung carcinoma (NSCLC) [1]. However, 20%-50% of patients experience recurrence and eventually die of recurrent lung cancer [2]. Adjuvant chemotherapy is recommended for patients with stage II and III lung cancer to prevent recurrence; however, it is not recommended for patients with stage IA of the disease. Further, even though the use of adjuvant chemotherapy for patients with stage IB lung cancer is controversial, it is recommended for patients with high-risk clinicopathological factors, such as lymphovascular invasion, visceral pleural invasion, and poor differentiation. However, using only clinicopathological factors to stratify the risk of tumor recurrence in patients with early-stage NSCLC to determine the course of adjuvant therapy is inadequate. To fully clarify the potential benefits of adjuvant chemotherapy or targeted therapy in patients with early-stage lung cancer, it is necessary to predict the risk of tumor recurrence using additional genomic alterations.
Epidermal growth factor receptor (EGFR) mutations are the most common driver mutations in East Asian patients with lung adenocarcinoma (40%-60%), and several potent EGFR–tyrosine kinase inhibitors (TKIs) have increased the median overall survival of patients with advanced-stage disease to longer than 2.5 years. However, not all patients benefit equally from TKI treatment owing to clinical and biological heterogeneity. Recently, the ADAURA clinical trial revealed that adjuvant treatment of resected stage IB-IIIA EGFR-mutant NSCLCs with third-generation EGFR-TKI osimertinib shows significantly better outcomes than placebo [3]. However, considering the high cost, adverse effects, and long treatment period associated with EGFR-TKIs, whether all patients with stage I NSCLC should be treated via this approach is still controversial. Thus, a strategy for the selection of patients at high risk of recurrence is necessary when considering adjuvant TKI therapy. Studies on predictive genomic markers of the benefits of adjuvant TKI therapy in patients with early-stage EGFR-mutant lung adenocarcinoma are limited [4]. Recent studies have focused on the risk stratification of advanced-stage EGFR-mutant NSCLC using nextgeneration sequencing (NGS) [5]. Further, several studies have been conducted to discover novel predictive biomarkers for the recurrence of resected early-stage NSCLC using various methods, such as gene expression profiling, quantitative reverse transcriptase–polymerase chain reaction, microRNA assays, and mass spectrometry [6-8]. Despite these efforts, the genomic biomarkers and mechanisms associated with recurrence of resected early-stage NSCLC are still unclear.
NGS allows the simultaneous evaluation of multiple genes and has recently been used in clinical practice to elucidate tumor biology and select targeted therapies to facilitate precision medicine strategies for patients with lung cancer [9]. Compared to traditional single-gene assays, NGS is a feasible and cost-effective method for diagnostic genomic profiling. However, it is unclear how the high volume of genomic alterations identified via NGS interact and how they can be used alongside EGFR mutation status and clinicopathological predictors to stratify the risk of tumor recurrence. To address this issue, we used large-panel NGS to analyze stage I EGFR-mutant lung adenocarcinoma specimens to investigate genomic alterations and oncogenic pathway co-alterations as well as their association with clinical outcomes.
Materials and Methods
1. Patients and samples
Patients with lung cancer who underwent complete tumor resection and were histologically confirmed to have stage I lung adenocarcinoma between 2005 and 2013 were screened for inclusion in this study. Of these patients, those with EGFR-mutant stage I adenocarcinoma were selected based on a review of electronic pathological reports and additional confirmation of EGFR mutation status using the PNAClamp EGFR Mutation Detection Kit (Panagene Inc., Daejeon, Korea) or the PANAMutyper EGFR Kit (Panagene Inc.), according to the manufacturer’s instructions. Electronic medical records were reviewed, and age, sex, smoking history, pathologic stage, lymphovascular invasion, visceral pleural invasion, and dates of tumor recurrence and death/last follow-up were collected. Patients with non-adenocarcinoma histology, insufficient tumor specimens for complete molecular analysis, and those who received neoadjuvant treatment were excluded. Pathological stages were reviewed and assigned according to the 8th American Joint Committee on Cancer (AJCC) criteria. Further, the International Association for the Study of Lung Cancer (IASLC) grades were determined according to previously described criteria [10]. This retrospective study was approved by the institutional review board (approval No. 4-2021-1181).
2. Targeted NGS and interpretation
DNA was extracted from formalin-fixed, paraffin-embedded whole tissue sections with more than 30% tumor cellularity using a QIAamp DNA kit (QIAGEN GmbH, Hilden, Germany) according to the manufacturer’s protocol. A TrueSight Oncology 500 panel (Illumina, San Diego, CA) was used to target 523 cancer-related genes. Further, libraries were sequenced on the NextSeq 550Dx platform (Illumina). Raw sequencing data were processed, and variants were identified using the Illumina bioinformatics pipeline (Illumina). Somatic mutations, including single-nucleotide variations, small insertions and deletions (indels), and copy number variations (CNVs) were also identified. Pathogenic and likely pathogenic somatic mutations with variant allele frequencies (VAFs) > 5% were regarded as significant and used for analysis. Fold changes above 1.5 or below 0.5 were regarded as CNVs. Furthermore, variant interpretation was based on recommendations from the Association for Molecular Pathology, the American Society of Clinical Oncology, and the College of American Pathologists [11].
The tumor mutation burden (TMB) scores (total variant number/megabase) were calculated according to the TrueSight Oncology 500 Local App Manual (Illumina). We also included single-nucleotide variants, insertions, and deletions in the coding region with a VAF between 5% and 90% and a read depth of 50×. However, we excluded certain variants that were annotated with ≥ 50 counts in the COSMIC database, ≥ 10 counts in normal population databases (gnomAD exome/genome, 1000 Genomes), or were present in the internal germline variant database. For the classification of samples based on TMB, we categorized samples with TMB scores below the median as ‘low TMB,’ while those with TMB scores equal to or above the median were classified as ‘high TMB.’
3. Number of pathway alterations
We evaluated 11 recognized signaling pathways using templates provided by The Cancer Genome Atlas PanCancer Atlas project [12]. The analyzed pathways included the receptor tyrosine kinase (RTK/RAS), Hippo, phosphoinositide 3-kinase (PI3K), transforming growth factor β (TGFβ), β-catenin/Wnt, Myc, p53, oxidative stress response/Nrf2, Notch, cell cycle, and DNA damage pathways. A tumor sample was considered ‘altered’ with respect to a specific pathway when one or more genes in the pathway contained a recurrent or known driver alteration. Additionally, the status of specific pathways was determined to be either altered or wild-type for each patient, and the number of pathway alterations (NPA) in each sample was calculated as the total number of altered pathways [13]. We classified samples with one or two pathway alterations as ‘NPA low,’ and those with three or more pathway alterations as ‘NPA high,’ and grouped them accordingly.
4. Statistical analysis
Clinical and pathological parameters were evaluated by performing the chi-square test or Fisher’s exact test for categorical variables and the Spearman rank correlation test for continuous variables. To assess prognostic values, we performed survival analyses. Recurrence-free survival (RFS) is the period from complete surgical resection to the time of the first documentation of recurrence or last follow-up, and progression-free survival (PFS) is the period from EGFR-TKI treatment to the time of progression or last follow-up, were analyzed using the Kaplan-Meier method, and differences were tested using the log-rank test via univariate analysis. Additionally, the Cox proportional hazards model was used for multivariate survival analysis. Statistical significance was set at p < 0.05. All statistical analyses were performed using the SPSS software ver. 26 (IBM Corp., Armonk, NY).
Results
1. Clinicopathological characteristics
Of 412 patients with stage I lung adenocarcinoma identified, 257 (62%) harbored EGFR mutations, and of these, 50 (19.5%) experience disease recurrence. All recurrent tumors (n=50) as well as randomly selected non-recurrent tumors (n=55) were further analyzed via NGS. The distributions of age, sex, smoking history, pathologic stage, and follow-up time of the 55 patients in the non-recurrent group were similar to those of the remaining 152 unselected patients (S1 Table). The median follow-up duration was 65 months (range, 7 to 126 months). The patient characteristics are summarized in Table 1, from which it is evident that the mean patient age was 63.8 years (range, 26 to 87 years). Further, 59 patients (56.2%) were female and 74 (70.5%) had never smoked. The mean number of pack-years smoked was 8.2 (range, 0 to 110), and the baseline pathological stage distribution was as follows: stage IA1, 8 (7.6%); stage IA2, 20 (19.0%); stage IA3, 35 (33.3%); and stage IB, 42 (40.0%). Furthermore, IASLC grades 1, 2, and 3 of the disease were observed in 20 (19.0 %), 60 (57.1%), and 25 (23.8%) patients, respectively.
2. Genomic characteristics
The L858R mutation in exon 21 was the most frequently observed EGFR alteration (53.3%), followed by exon 19 deletions (E19del) (38.1%) and other alteration types (8.6%). The co-occurring genetic alterations in all the 105 patients included are shown in Fig. 1 and S2 Table. Among the co-occurring mutations, TP53 mutations were the most frequently observed (n=17, 16.2%), followed by RBM10 mutations (n=9, 8.6%). Further, the most frequent co-occurring CNVs were: RPS6KB1 amplification, EGFR amplification, MDM2 amplification, CDK4 amplification, and MYC amplification observed in 25 (23.8%), 22 (21.0%), 12 (11.4%), 10 (9.5%), and 10 (9.5%) tumors, respectively.
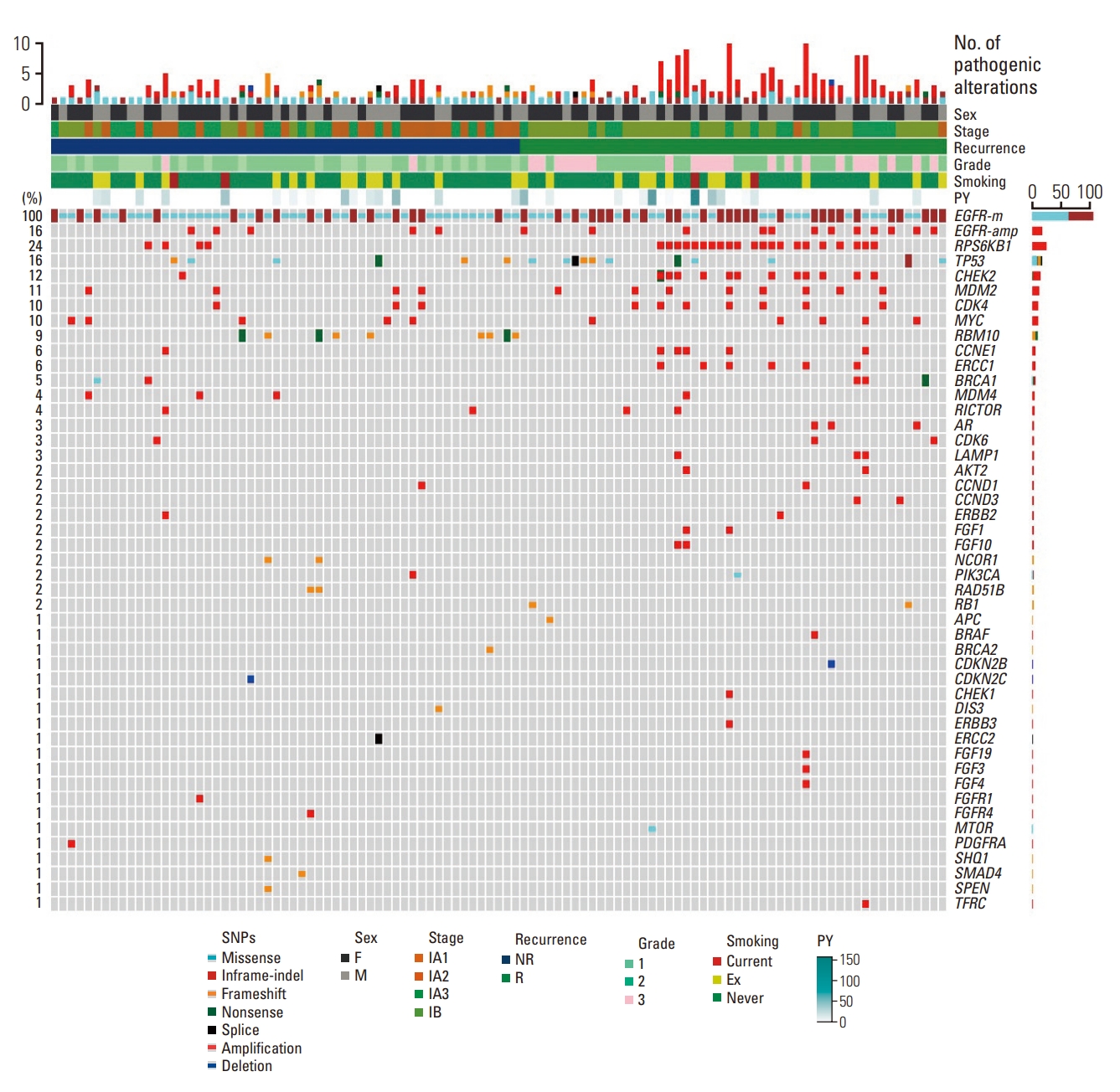
Genomic landscape of stage I EGFR-mutant lung adenocarcinoma according to recurrence status. EGFR, epidermal growth factor receptor; NR, not recurred; PY, pack-years; R, recurred.
The distribution of pathway alterations is shown in Fig. 2. From this figure, the p53 pathway was identified as the most frequently altered pathway (n=39, 37.1%), followed by the PI3K pathway (n=29, 27.6%), cell cycle (n=22, 20.9%), and DNA damage (n=15, 14.2%). The NPA distributions were as follows: NPA1, 40 (38.1%); NPA2, 32 (30.5%); NPA3, 24 (22.9%); and NPA4, 9 (8.5%). Additionally, the median NPAs were 2 (range, 1 to 4) for all patients, 2.5 (range, 1 to 4) for the recurrence group, and 1.5 (range, 1 to 3) for the non-recurrence group.
The median TMB was 4.7 per megabase considering all the patients. We also observed a high TMB (≥ 4.7) in 56 patients (53.3%). The median TMB was 5.7 (range, 0.8 to 40.1) in the recurrent group, and 3.9 (range, 0.8 to 49.8) in the non-recurrent group.
3. Prognostic factors of RFS in all patients
In the univariate analysis, pathological stage, IASLC grade (Fig. 3A), lymphovascular invasion, visceral pleural invasion, TP53 mutation, NPA, PI3K pathway, p53 pathway, and cell cycle pathway (Fig. 3B) were significantly associated with a worse RFS. The hazard ratio (HR), 95% confidence interval (CI), and p-values are shown in Table 2. Additionally, RPS6KB1 or EGFR amplifications were associated with a worse RFS (Table 2). A high TMB was also associated with poor RFS (p=0.004).
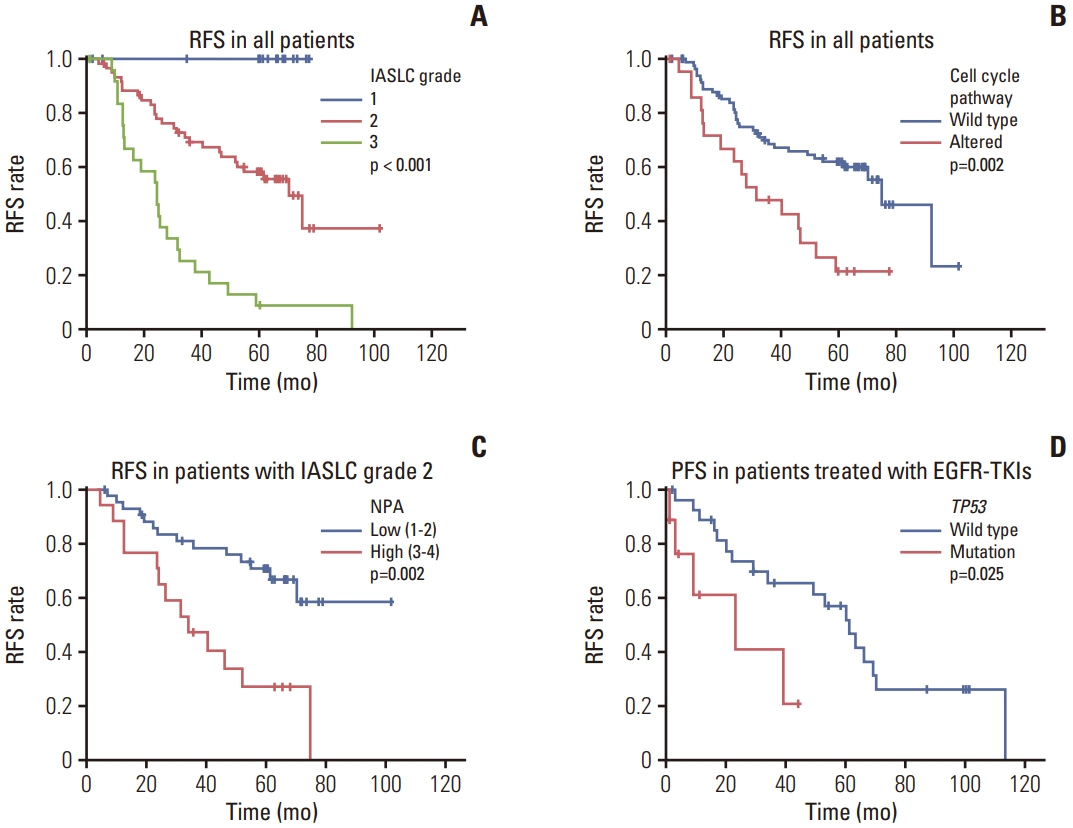
Survival curves based on IASLC grades and genomic alterations. (A) RFS of all patients based on IASLC grades. (B) RFS for all patients based on cell cycle pathway alterations. (C) RFS for patients with IASLC grade 2 EGFR-mutant lung adenocarcinoma based on NPA. (D) PFS for patients treated with EGFR-TKI after recurrence based on TP53 mutations. EGFR, epidermal growth factor receptor; IASLC, International Association for the Study of Lung Cancer; NPA, number of pathway alterations; PFS, progression-free survival; RFS, recurrence-free survival; TKI, tyrosine kinase inhibitor.
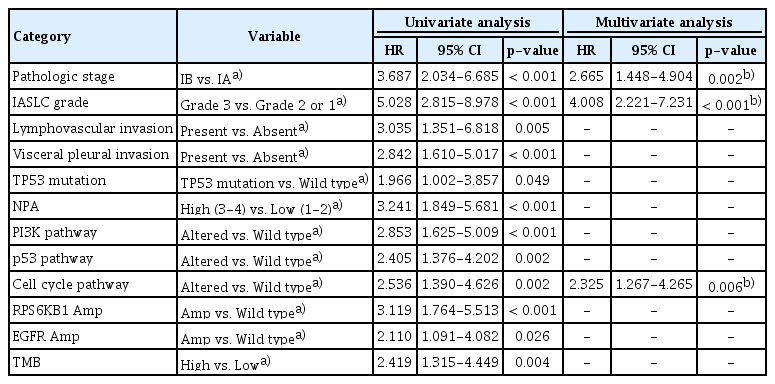
Prognostic factors for recurrence-free survival according to univariate and multivariate analyses involving all patients (n=105)
Multivariate Cox regression analysis revealed that independent risk factors for RFS included a higher pathologic stage (HR, 2.665; 95% CI, 1.448 to 4.904; p=0.002), higher IASLC grade (HR, 4.008; 95% CI, 2.221 to 7.231; p < 0.001), and an altered cell cycle pathway (HR, 2.325; 95% CI, 1.267 to 4.265; p=0.006) (Table 2).
4. Prognostic factors of RFS in patients with IASLC grade 2
No disease recurrence was observed in patients with grade 1 of the disease, and as expected, patients with grade 2 disease (n=60) showed an intermediate prognosis among the three patient groups according to the IASLC grading system (Fig. 3A). Additionally, univariate analysis showed that pathological stage, visceral pleural invasion, NPA (Fig. 3C), PI3K pathway, cell cycle pathway, and RPS6KB1 amplification were significantly associated with a worse RFS. The HR, 95% CI, and p-values are shown in Table 3.

Prognostic factors for recurrence-free survival according to univariate and multivariate analysis in patients with IASLC grade 2 (n=60)
Multivariate Cox regression analysis further revealed that independent risk factors for RFS included a higher pathological stage (HR, 3.327; 95% CI, 1.465 to 7.557; p=0.004) and a higher NPA (HR, 3.271; 95% CI, 1.497 to 7.147; p=0.003) (Table 3).
5. Prognostic factors of PFS in patients treated with EGFR-TKI after recurrence
Among patients with recurrence who underwent EGFR-TKI treatment (n=37), those with co-occurring TP53 mutations showed shorter PFS periods (p=0.025) (Fig. 3D). Other factors did not show statistically significant associations between PFS and the EGFR-TKI treatment.
Discussion
Here, we evaluated the prognostic implications of co-occurring genetic and pathway alterations in patients with surgically resected stage I EGFR-mutant lung adenocarcinoma. Thus, we observed that: (1) cell cycle pathway alteration and IASLC grade were independent poor prognostic factors in stage I EGFR-mutant adenocarcinoma, (2) a higher NPA was an independent prognostic factor in IASLC grade 2 EGFR-mutant adenocarcinoma, and (3) TP53 mutation was associated with a poor response to EGFR-TKI therapy after recurrence in completely resected stage I EGFR-mutant adenocarcinoma.
The prognostic factors associated with disease recurrence in early-stage lung adenocarcinomas after complete resection have been investigated in previous studies. Among these, it has been consistently reported that a histologically high-grade pattern is associated with a poor prognosis [14-16]. As an extension of these findings, the IASLC pathology committee proposed a grading system for invasive non-mucinous lung adenocarcinoma [10], and this grading system has been validated in another cohort [17-19]. Thus, as expected, IASLC grade was identified as an independent prognostic factor for RFS in patients with stage I EGFR-mutant cancers.
The use of NGS panel testing has become increasingly common in clinical practice, particularly for patients with advanced lung cancer. This test primarily provides information on targetable genetic alterations [9]. In addition to targetable drivers, the presence of co-occurring genetic alterations can affect cancer biology, microenvironmental interactions, and therapeutic outcomes [20]. Thus, comprehensive genomic profiling of cancer-related genes has become important, and large-panel NGS, which offers the possibility to detect mutations in tumor suppressor genes and CNVs, is frequently used in clinical settings. In this study, mutations or amplifications in the genes, TP53, or RPS6KB1 were associated with a worse RFS. These findings confirmed the effect of co-occurring genetic alterations on clinical outcomes, even in stage I lung cancers, including EGFR oncogene-driven adenocarcinomas.
In addition to the gene-centric approach described above, the pathway-centric approach can also provide additional clinical information [13]. In this study, we investigated 11 oncogenic pathway alterations as well as NPA and observed that certain co-occurring pathway alterations (PI3K, p53, and cell cycle) and a high NPA (≥ 3) were associated with a worse prognosis. Via multivariate analysis of the study population, we also observed that an altered cell cycle pathway and a high NPA were independent prognostic markers associated with poor RFS. This possibly indicated that the pathway-centric approach offers the possibility to more effectively identify patients at high risk of recurrence as well as candidates for adjuvant therapy following lung adenocarcinoma resection. Similar findings using the pathway-centric approach were obtained in a study on resected lung adenocarcinoma [13]. In this previous study, NPA was identified as an independent risk factor for a poor RFS and cell cycle, Hippo, TGFβ, and p53 pathway alterations were also found to be associated with a poor RFS [13]. Another recent study found that higher NPA, TMB, and CNVs are linked to high-grade histologic patterns and worse clinical outcomes in stages I-III lung adenocarcinoma [21].
In this study, TP53 mutations, which were the most frequently observed co-mutations, were associated with a worse RFS. Previous studies have suggested that TP53 alterations may be associated with treatment resistance and shorter survival in patients with EGFR-mutant lung cancer [22-24]. Further, it was recently suggested that TP53 mutations influence clinical outcomes by facilitating genomic instability and the acquisition of additional co-occurring driver events in early-stage EGFR-mutant lung cancer [25]. Furthermore, TP53 mutations are associated with faster resistance evolution independent of the resistance mechanism after TKI treatment in advanced-stage EGFR-mutant lung cancer [26]. In this study, TP53 mutations showed an association with a poor PFS following EGFR-TKI treatment in patients with recurrent disease after complete surgical resection of stage I disease.
Our results also indicated that RPS6KB1 and EGFR amplifications were significantly associated with a worse RFS in stage I EGFR-mutant lung adenocarcinoma. Among these, RPS6KB1 amplification was the most frequently observed (in 23.8% of cases). Reportedly, the RPS6KB1 gene encodes a member of the ribosomal S6 family of serine and threonine kinases. The encoded proteins then respond to mTOR signaling to promote protein synthesis, cell growth, and proliferation. Additionally, RPS6KB1 amplification has been reported in approximately 10% of breast cancers and is associated with poor clinical outcomes [27]. Hyperphosphorylation of RPS6KB1 has recently been correlated with poor clinical outcomes in patients with NSCLC after surgical excision [28]. Notwithstanding, further studies on RPS6KB1 amplification in a larger cohort with functional validation are needed to confirm its biological and clinical significance.
Surgically resected lung adenocarcinoma is graded according to the IASLC-proposed grading system [10]. Real-world data show that grade 2 tumors constitute the largest proportion (> 50%) of lung adenocarcinomas [19]. Further, grade 2 tumors, formerly intermediate-grade adenocarcinoma, can be heterogeneous tumors with various clinical outcomes [29]. In the present study, we examined the significance of co-occurring genetic and pathway alterations in grade 2 tumors. In particular, NPA was identified as an independent prognostic factor for a worse RFS. This indicated that genomic analysis of tumors with an intermediate prognosis could provide additional information for predicting clinical behavior in lung cancer.
Systemic targeted or immunotherapies have been increasingly used in adjuvant or neoadjuvant settings for patients with resectable early-stage lung cancer [30]. Accordingly, the early determination of genomic and immunological information for early-stage lung cancer is becoming increasingly important [31]. NGS, which is intensively performed for advanced-stage cancers, is expected to expand the indications for early-stage cancers. We believe that understanding the detailed genetic and pathway alterations in early-stage lung cancer may facilitate the development of new therapeutic strategies to improve patient care, such as risk stratification and the development of novel candidates for combination therapy, even in patients with stage I EGFR-mutant lung adenocarcinoma.
Our study had several limitations. First, this was a retrospective study; therefore, the possibility of selection bias cannot be ruled out. Second, we used only DNA sequencing data. Genes that were epigenetically silenced were excluded from the analysis; transcriptome analysis can provide additional information on high-risk factors [24]. Third, we sequenced only a portion (27%) of the non-recurrent tumors owing to resource limitations. Although the selected non-recurrent group showed a similar distribution of clinical characteristics as the remaining unselected patients, there could be a selection bias. The non-recurrent group was predominantly composed of patients with grades 1 and 2 tumors. Additionally, in this study, we mainly focused on recurrence-associated genomic factors. Due to the limited number of cases in our study, we did not perform detailed analyses regarding grade-associated genomic alterations. It is worth noting that previous reports have described associations between high-grade tumors and their genomic findings [21,32].
In conclusion, the results of this study suggested that large-panel NGS with genomic and pathway-centric analysis strategies may provide valuable information for the risk stratification of patients with resected stage I EGFR-mutant lung adenocarcinoma. In particular, cell cycle alterations and high NPA were independently associated with a worse RFS, and TP53 mutations were associated with poor response to EGFR-TKIs after recurrence. These results may facilitate risk stratification and the development of new therapeutic strategies for patients with early-stage EGFR-mutant lung cancer.
Electronic Supplementary Material
Supplementary materials are available at Cancer Research and Treatment website (https://www.e-crt.org).
Notes
Ethical Statement
This study was approved by the Institutional Review Board (local IRB number: 4-2021-1181). As this was a retrospective study, the IRB waived the requirement for informed consent due to the study design.
Author Contributions
Conceived and designed the analysis: Lee JS, Kim EK, Kim KA, Shim HS.
Collected the data: Lee JS, Kim EK, Kim KA, Shim HS.
Contributed data or analysis tools: Lee JS, Kim EK, Kim KA, Shim HS.
Performed the analysis: Lee JS, Kim EK, Kim KA, Shim HS.
Wrote the paper: Lee JS, Kim EK, Kim KA, Shim HS.
Conflicts of Interest
Conflict of interest relevant to this article was not reported.
Acknowledgments
This research was funded by the Basic Science Research Program through the National Research Foundation of Korea (NRF) funded by the Ministry of Education (grant number NRF-2018R1D1A1B07047811) and the National Research Foundation of Korea (NRF) grant funded by the Korea government (MSIT) (grant number 2022R1A2C1009364).